Blog
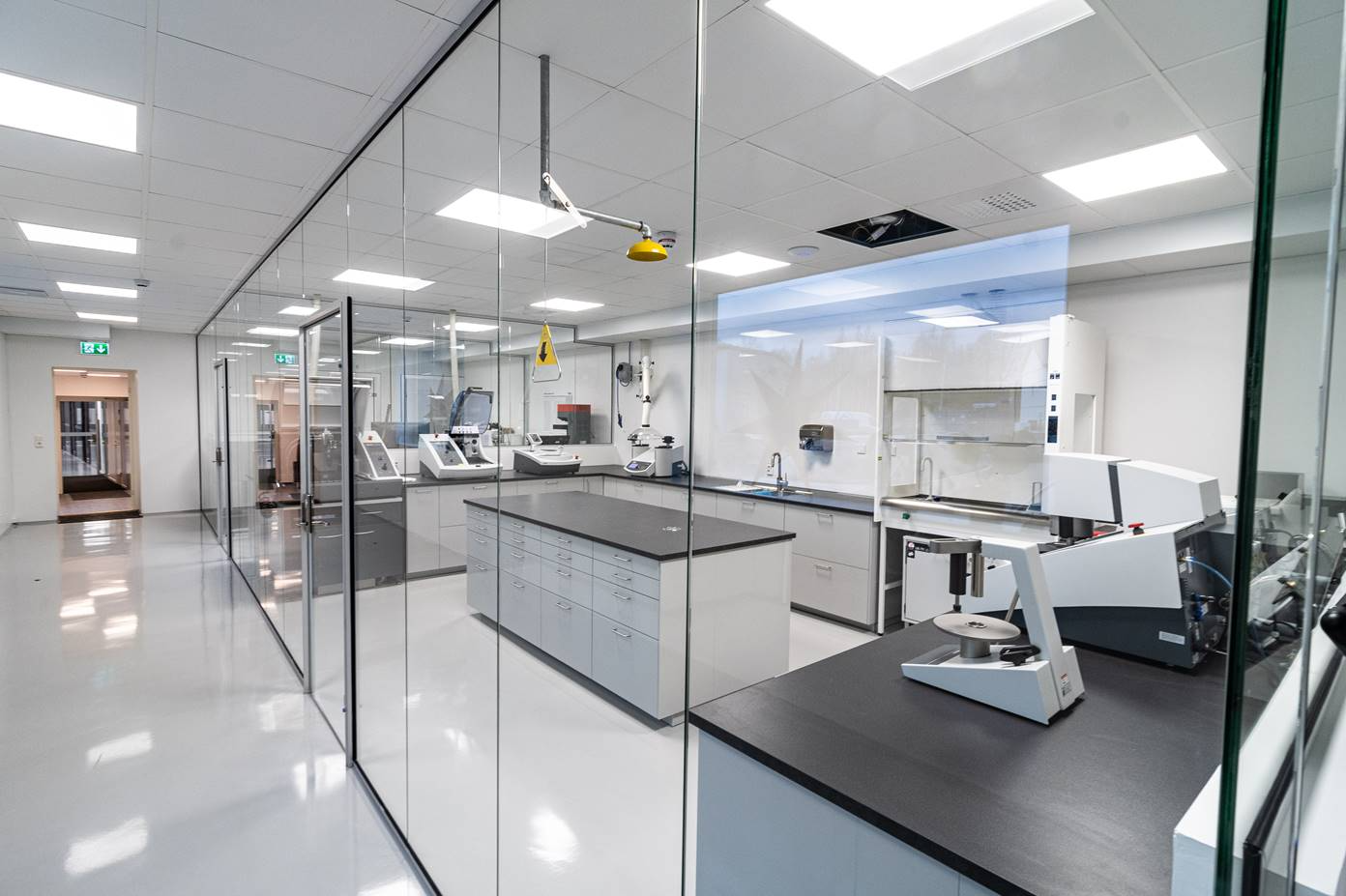
Gaussian, top hat or donut? Part I: Exploring the effect of beam shape on the productivity and density of additively manufactured AlSi10Mg.
The EU project InShaPe: Green Additive Manufacturing Innovative Beam Shaping and Process Monitoring aims at increasing the speed of laser powder bed fusion (PBF-LB/M) metal additive manufacturing (AM), while simultaneously reducing its cost and environmental impact. Three different materials, namely Inconel 718, CuCrNb and AlSi10Mg, have been investigated within the project.
PBF-LB/M is currently considered the most mature method within AM technologies. In this technique, a layer of metal powder of between 30 to 100 µm in thickness is deposited on a build platform and subsequently a laser is used to selectively melt certain areas, according to a digital model. This layer-by-layer process is repeated to produce near- or net shaped components.
The InShaPe beam shaping technology is expected to enhance the productivity of PBF-LB/M and concurrently make the process more adjustable and robust, as it can aid in heat distribution control within the process zone, which broadens the parameter window for material processing. Furthermore, this novel technology holds great potential for microstructural tailoring, so that specific sections of a component are customized using appropriate processing parameters. The beam shape has almost unlimited possibilities, as illustrated in Figure 1.
Figure 1. Beam shapes that can be achieved with the InShaPe system. Left: Gaussian beam shape. Right: Donut, Top hat and other novel beam shapes. Image: courtesy of EOS.
Although some beam shapes, e.g., donut, have shown promising results reducing defects and expanding the processing parameters window to produce AlSi10Mg1, no parameters have been established and optimized for laser powers above 800 W. Furthermore, a systematic evaluation of the effect of beam shapes on both density and productivity for the manufacturing of AlSi10Mg is a crucial step for the future development and optimization of this promising technology.
In February 2024, the initial trials were commenced in a joint effort between AMEXCI AB and EOS GmbH to investigate the effect of different beam shapes on the PBF-LB/M process build rate and porosity for AlSi10Mg. An EOS M 300-4 prototype with an integrated beam shaping system and maximum laser power of 1.3 kW was used.
In total, five distinct designs of experiments (DOEs) were conducted using layer thicknesses of 60 µm, 90 µm and 120 µm. In these DOEs, 10 mm cube specimens were produced using different combinations of beam spot size, hatch distance, laser power, laser speed and beam shapes. The specimens produced in one of the trials is shown in Figure 2.
Figure 2. Left: 10 mm cube specimens produced with 90 µm layer thickness. Right: AMEXCI materials lab used for sample analysis. Images: Amit Saini/AMEXCI, AMEXCI Materials Lab Newsroom – AMEXCI.
The density of 180+ samples was evaluated using Archimedes principle, as shown in Figure 3. Based on the measured densities and the theoretical build rates, several samples from each layer thickness were selected for further porosity investigations with optical microscopy. The following Figure 3 and Figure 4 showcase how preparation of specimens for optical microscopy evaluation has been conducted at the materials lab.
Figure 3. Density evaluation using Arquimedes principle and samples’ sectioning for optical microscopy investigations. Images: Merve Canalp and Victor Pacheco/AMEXCI.
Figure 4. Preparation of selected specimens for optical microscopy evaluation through grinding and polishing. Images: Merve Canalp/AMEXCI.
The evaluation of selected specimens with optical microscopy has been recently finalized at AMEXCI Material Lab facilities. Based on the results, the next trials will be designed and implemented to establish parameters that provide both dense parts and high productivity in the manufacturing of AlSi10Mg with PBF-LB/M.
The development of parameters with a good balance between density and productivity is an essential step before upscaling and demonstrating the beam shaping technology for AlSi10Mg. This will be done in the future using AMEXCI’s use-case, which consists of a cylinder head for a chain saw. News and updates on the developments of this exciting project will follow in future blog posts. Stay tuned!
1 Tim Marten Wischeropp, Hussein Tarhini, Claus Emmelmann; Influence of laser beam profile on the selective laser melting process of AlSi10Mg. J. Laser Appl. 1 May 2020; 32 (2): 022059. https://doi.org/10.2351/7.0000100.